MRO and NERC
Richard Burt is Senior Vice President and Chief Operating Officer, Midwest Reliability Organization (MRO).
Mark Lauby is Senior Vice President and Chief Engineer, North American Electric Reliability Corporation (NERC).
The U.S. economy and nearly four hundred million North Americans depend on a reliable supply of electricity to support their businesses, communities, and quality of life. As our economy becomes more electrified, the changing resource mix, decarbonization of the grid, and increasingly extreme and unpredictable weather place an even greater emphasis on the need for a resilient and reliable bulk power system (BPS).
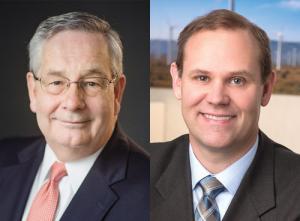
In this period of unprecedented change, how certain are we that energy will be available to serve load at any given time? One thing is certain — with the changing resource mix, we are a lot less sure than we once were, regardless of the time frame being assessed (seasonal, long-term, or operational).
The previous method for assessing resource adequacy may be obsolete. We need to rethink how we understand and quantify generating capacity, energy supply, and how load is served.
The need for a new energy assurance method is reinforced by observations from previous extreme hot and cold weather events, and forward-looking assessments of the grid. All of these point to energy assurance being the most significant reliability challenge the electric industry now faces. There are many facets of energy assurance, such as the changing resource mix, variable resources, behind-the-meter generation, transmission deliverability, and shrinking reserve margins.
Background
Historically, the assessment of BPS resource adequacy focused on capacity over peak time periods. This assessment concentrated on capacity reserve levels compared to peak demand, because resources were generally dispatchable and available when needed, except in the instances of unit outages and de-rates.
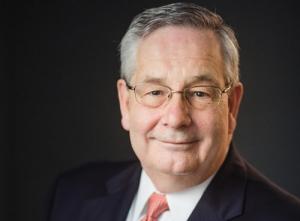
Reserve margins historically were planned so that deficiency in capacity to meet daily peak demand loss of load expectation (LOLE) or loss-of-load probability (LOLP) occurred no more than one event in ten years.
Reserve margins are calculated from probabilistic analysis using generating unit forced outage rates based on random equipment failures derived from historic performance. Those forced outages were driven mostly by independent random equipment failures. The targeted level has been based on daily peaks, rather than hourly energy obligations.
Additional insights were traditionally gained by also calculating loss-of-load hours and expected-unserved-energy based on the mean-time-to-repair averages for each unit. Review and clarification of such traditional metrics is needed to better understand these assumptions and put forward additional meaningful measures that support and reflect the new operating paradigm for capacity and energy delivery.
Key Assumption
A key assumption in the application of current methods is that fuel is available when capacity is required to provide the requisite energy. This approach reflects an operating environment where, fuel availability was generally assured with either long-term fuel contracts (commodity plus transportation capacity), on-site storage such as oil, coal, and reservoir-based hydro, and/or with required periodic and predictable fuel replacement such as nuclear.
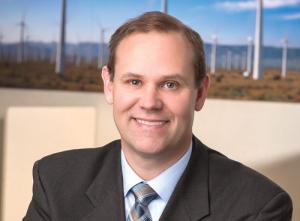
Now, diverse, dispatchable resource technologies, and capacity from other technologies could mitigate the impacts if fuel for one resource type became unavailable. Going forward, the provision of fuel flexibility will remain an issue at least until large-scale electric storage or other solutions are available to attenuate the fuel draw requirements to support steep ramp rates characterized by resources such as solar generation.
As the resource mix transforms, it is apparent that energy assurance is more susceptible to weather conditions and the availability of variable generation. Impacts from long-term and widespread extreme temperatures and/or droughts can dramatically affect the availability of capacity required to produce the energy needed to meet consumer demand.
Extreme weather conditions can have a higher and broader impact on the BPS than individual system outages that are due to random equipment failures, as large amounts of resources with a common fuel source are put at risk at the same time.
As generation is lost, greater demand is placed on those generators that are operating, which may not be able to provide sufficient amounts of energy to meet the needs of the consumer. This can result in large amounts of load shedding to preserve the reliability of the bulk power system.
Until recently, capacity was determined using conventional generation resources such as fossil fuel and nuclear power plants that provided the resources needed to deliver energy, reliability services, and system flexibility.
The transforming resource mix has introduced a level of uncertainty to this construct, and now each component must be addressed separately in order to understand the characteristics of the capacity mix, and the ability to support the reliable operation of the BPS.
Changing Times
The era of fuel on demand is ending. Transitioning from coal and nuclear resources to wind, solar, natural gas (including dual fueled), and hybrid resources creates a more complex scenario wherein fuel assurance and forward energy supply planning becomes increasingly important and much more complicated.
Generating capacity alone is not sufficient to ensure the reliable operation of the BPS. Increased contribution of renewable energy places a higher emphasis on the on-call availability of capacity to supply energy to serve load.
Production flexibility from these balancing resources is already important and will become critically important in the future. Operational uncertainty is increasing due to the types of, and conditions under which, energy, and by implication, fuel, is available or acquired.
Some examples of these uncertainties are resources solely dependent on the availability of wind and solar. These resources are similar to run-of-river hydro plants in that they have no fuel storage capabilities and are dependent on real-time weather conditions.
This results in energy output being a fraction of the energy availability assumed from full nameplate capacity planning and reserve margins. These also include distribution level resources and flexible load programs that may introduce additional volatility into energy forecasts.
Layered into operational uncertainty is that some areas' natural gas fueled resources may, depending on the contract for fuel acquisition, be subject to fuel curtailment or interruption during peak fuel demands.
Additionally, natural gas pipeline design and the way in which generators interconnect with the pipeline can vary, resulting in significantly different impacts to the generator and the BPS under pipeline disruption scenarios. In some areas, variable energy resources require that there are sufficient flexible energy resources available to quickly respond to offset ramping requirements.
The impacts can be mitigated with the supply and geographical diversity from renewable and smaller distributed resources. However, this resource uncertainty is already causing many system operators to consider scheduling, as well as optimization and commitment of resources over a multi-day timeframe.
Replacing the existing generation fleet with energy-limited resources requires industry to consider both capacity requirements and energy sufficiency, and by extension, fuel availability. Even if sufficient capacity is available, a level of certainty in the delivery of fuel is required to ensure that energy is available to support demand.
Conditions in California have demonstrated that when solar becomes a significant resource, the flexibility of the natural gas system (generating plant ramping capability plus pipeline flexibility to support needed ramp rates) also becomes a key planning consideration. This issue came into focus with the limitations placed on the Aliso Canyon natural gas storage facility that caused operational challenges in ensuring adequate pipeline pressure was available to support the late afternoon ramp.
Understanding Components of Energy Sufficiency
Ensuring energy sufficiency is challenging due to the many variables involved in the equation. The most problematic aspect is that it requires revisiting some of the common assumptions that are fundamental to how we plan and operate the system — things we learned in Power Systems 101:
Is capacity a relevant concept anymore?
What is the value of using reserve margins for planning and operations, as currently calculated?
What does a transmission system even look like when it is no longer an electron interstate between large baseload generators and load centers?
Is it possible that the most critical n-1 contingency is actually loss of a natural gas pipeline and not a generation or transmission element?
What assumptions are we using in 50/50 and 90/10 scenarios and are they really 50/50 and 90/10?
For illustration, in the last question, note that forecasts cannot precisely predict the future. Instead, many forecasts report probabilities with a range of possible outcomes. For example, each demand projection can represent the expected midpoint of possible future outcomes. This means that a future year's actual demand may deviate from the projection due to the inherent variability of the key factors that drive electricity use, such as weather. Namely, there is a 50 percent probability that actual demand will be higher than the forecast midpoint and a 50 percent probability that it will be lower (50/50 forecast)
Three Time Frames
In today's environment, grid operators must consider energy sufficiency requirements that need to be planned and available over three time frames:
Mid- to Long-Term Planning: When undertaking mid- to long-term planning for resources to support the system in the one-to five-year time frame, grid planners must ensure that sufficient amounts of energy are in place such that appropriate options are available to acquire needed energy to meet demand and flexibility requirements for reliably operating the BPS throughout all seasons of the year and need to account for extreme weather scenarios as well.
Review of traditional approaches and metrics is required to identify and put forward advances needed to support energy sufficiency; this includes considering fuel contract types, dual-fuel requirements, hybrid resource requirements, projected emission limitations, early unit retirements, forced outage uncertainty, and scenario analysis of wind, solar, and droughts, etc., under normal and n-1 scenarios.
Operational Planning: When evaluating the operational planning timeframe (one day to one year), grid operators must ensure that sufficient units are available with the ability to provide the needed energy both to meet demand and offset potential ramping requirements.
Electrical energy production measurements need to reflect contracts in place, dual-fuel availability, unit maintenance, and fuel supply (such as liquefied natural gas) levels, as well as barge and other transportation requirements for short-term turnaround to re-supply as needed.
Fuel assurance must support energy being available for defined scenarios. The operational planning time frame includes forecasting of variable renewable resources, and the forward scheduling, optimization, and commitment of power system resources to produce the needed energy to meet forecasted demand, which in turn leads to the scheduling, optimization, and commitment of the required fuel availability.
Real-Time Operations: When evaluating the real-time operations timeframe (zero to one day), grid operators must have situational awareness of energy sufficiency to ensure sufficient amounts of energy, reliability services, and ramp flexibility are available from existing resources.
This assessment includes fuel contract status, start-up time, unit maintenance, dual fuel availability, etc., of scheduled resources to ensure they are available to cover potential system contingencies, including ramping requirements, while meeting real-time demand.
In order to achieve this, there is a greater need for real-time and near-term situational awareness of fuel delivery systems (such as natural gas) and a greater dependency upon generation forecasts, particularly for generation with variable fuel supplies (such as solar and wind).
Analysis Requirements
The ability to model and address fuel limitations or shortages in BPS planning is a critical part of system planning and operations. Considering this, there is a need for improved models, as well as the required data and information to support planning improvements that ensure the continued reliable operation of the BPS. The following should be considered during model development and planning:
Identify Energy Limitations and Constraints. Every generator has some level of energy limitation: Solar resources and the availability of the sun's irradiance; Hydro-resources limited by the amount of water stored behind dams or run-of-river capacity; Natural gas resources limited by the transport capability of the pipeline system under normal and outage conditions as well as response capability; Dual fuel resources limited by the amount of on-site back-up fuel plus replenishment capability; and Coal resources limited by transportation needs, as well as cold and moisture impacts on reserve piles.
All resources are limited by forced outages (and partial outages) due to thermal stresses, equipment failure, and, in some cases, emission allowances and discharge water temperature values.
For example, fossil-fueled resources may experience energy limitations due to emission limitations, which are expected to increase over time. In addition, limitations can also be caused by transmission maintenance that inhibits energy deliverability. To further complicate analyses, market rules are in place to reserve limited-energy resources for a later time, optimizing the overall solution.
Identify the Tools and Methods Needed: For the mid- to long-term planning, operational planning, and operations time horizons, tools and methods are needed to identify the right mix of resources to ensure sufficient amounts of energy are available to serve demand at all times adequate ramping capabilities exist, and essential reliability services are available.
In addition, in organized markets, market-based incentives or rules, tariff changes, and other market tools need to be investigated. For example, some jurisdictions have evolved to performing eight thousand seven hundred sixty stochastic simulations to assess hourly levels of risk.
Some jurisdictions also have established locational, flexible, capability, and performance requirements into their resource adequacy programs. Reviewing existing tools and methods already developed, identifying any gaps, and providing guidance to address the gaps will support creation of systems that will have sufficient amounts of energy for the reliable operation of the BPS.
Conduct Loss-of-Load Assessments: The system must be planned (in both the mid-to long-term and operations planning time horizons) to provide a set of options to the operator so sufficient amounts of energy are available for the reliable operation of the BPS throughout all seasons of the year.
Energy limitations need to be incorporated into the electric power resource adequacy models to more accurately estimate key adequacy metrics, such as loss‐of‐load expectation, loss‐of‐load hours, and expected unserved energy. As the applications of electricity grows in North America, the impact of lost load will further increase, and as a result, the value of energy assurance to serve load will grow in importance.
Furthermore, as microgrid developments increase, assessment of contributions to reliability and consequences of energy sufficiency needs to be more fully understood. An important feature of integrating these suggested analyses with existing tools is the ability to incorporate operational solutions into the planning models. For example, incorporation of demand response, voltage reduction, and public appeals would be valuable.
By including cross-energy sector study results from energy limitations, such as fuel or pipeline infrastructure limitations, into probability‐based resource adequacy models, an accurate representation of risk can be quantified and then translated into risk‐based planning solutions.
Cross-energy sector studies should include agreed upon study criteria between the sectors on what it means to be reliable and what the implications are on system resilience. This is important, as one sector may have a view of reliability that does not translate into other dependent sectors.
For example, should sustaining the loss of a large natural gas storage field be considered a credible event impacting reliability of the BPS that should be addressed by both the natural gas and electric sectors?
Additionally, agreed upon contingencies impacting fuel transportation or severe weather event scenarios that impact multiple energy sectors are necessary. This analysis can be used for all time frames, incorporating more granular information as the system approaches the operations time frame.
Appropriate reliability metrics and criteria for the three time frames must be developed as the degree of uncertainty in assumptions varies across each time frame. Study is needed to determine if the same or different metrics are required when the three time frame assumptions have varying risk profiles.
Energy assurance is a difficult challenge, but the process of solving this complex problem will undoubtedly be exciting and transformative. By working together, we can meet these challenges and develop the solutions necessary to ensure the continued reliable operations of the bulk power system, while still maximizing the integration of resources that meet the goals of a decarbonized society.